Microscopic Robots Revolutionizing Multiple Fields
Researchers at Penn State have made a significant breakthrough in understanding how microscopic robots behave in tight spaces, opening up new possibilities for advancements in health, technology, and environmental science. Led by Stewart Mallory, assistant professor of chemistry and chemical engineering, the team has been studying active matter, specifically the collective behavior of self-propelled microscopic particles.
Unlocking the Secrets of Single-File Diffusion
The researchers focused on a phenomenon known as single-file diffusion (SFD), where particles are trapped in narrow channels and cannot pass each other. Using Brownian dynamics simulations, they discovered that the movement of active particles in such conditions initially appears ‘ballistic’ but eventually slows down and follows typical SFD behavior. Crucially, they found a direct link between the mobility of these particles and the compressibility of the system.
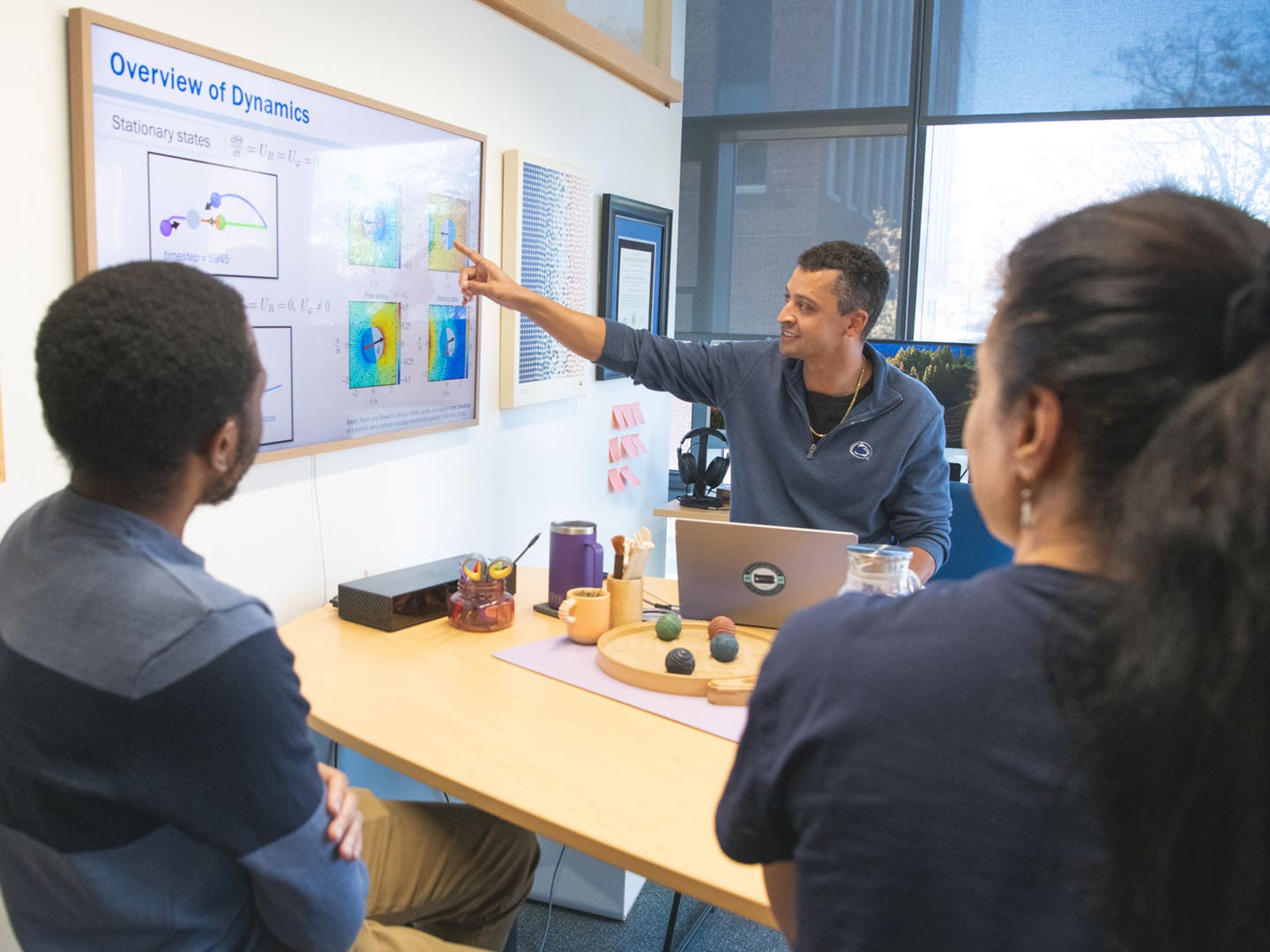
This advancement is key for real-world applications such as guiding micro-robots through blood vessels. By understanding exactly how long it takes for drug-delivering particles to reach their target, scientists can design better therapies that are faster and more reliable. The study’s findings have been published in The Journal of Chemical Physics.
Broader Implications of Active Matter Research
Mallory’s work has also shed light on the connection between micro and macro worlds. He notes that phenomena like ‘phantom traffic jams’ are examples of single-file dynamics in daily life, where small changes in speed or distance between cars can build up into major jams. This connection underscores the broad relevance of active matter research, with lessons learned from tiny swimmers potentially offering insights into everything from transportation to crowd control.
Tuning the Movement of Microswimmers
The research team has also made progress in controlling the movement of Phoretic Janus particles, another type of self-propelled nanoparticle. By adjusting the surface chemistry of these particles, researchers can guide how they move in response to specific chemical signals. This is crucial for real-world environments where many particles interact and influence each other’s paths.
Building New Materials and Healing the Environment
One of the most exciting frontiers for active matter lies in materials science and environmental cleanup. Mallory’s research shows that self-propelled particles could be used for self-assembly, creating new materials by designing nanoparticles that can move and arrange themselves into desired patterns. Additionally, active matter could help heal the planet by breaking down microplastics or detecting and targeting cancer cells.
As science delves deeper into the world of active matter, the results could transform how materials are made, diseases are treated, and the environment is restored. The power of the small may soon change the very fabric of our world.